The role of Mechanics in governing key biological processes at the cell scale is becoming increasingly apparent. At the triple point between biology, biophysics, and mechanics, Mechanobiology is emerging as a new, thriving research field, that is attempting to understand Biology from the perspective of Mechanics. And while the key role played by bio-chemical regulation in molecular cell biology is undisputed, the importance of forces and stresses in determining how cells function is becoming more and more widely recognized. Forces control shape and motion of the cells. But also how cells decide when and how much to differentiate (e.g., depending on the stiffness of the substrate or of the surrounding extracellular matrix), and where cells migrate to (mechano-sensing). Unveiling the details of this mechano-transduction opens the way to understanding key biological process at the cell scale. In addition, forces define much of the organization of the cells, hence they are important at the tissue and organ level, and are being used to drive artificially grown tissues in tissue engineering. Finally, many pathologies such as atherosclerosis, cancer, neurological and developmental deseases can be traced to abnormal mechanics. Cell motility provides one concrete example where Mechanics is shaping our understanding of key biological processes. Depending on whether the surrounding medium is a fluid or a solid (a matrix or a substrate), cells move either by swimming or by crawling. Mechanics is the natural tool to estimate the forces cells need to exert and the power to spend in order to move as we see them moving. Quantitative analysis of swimming of unicellular organisms, in which experimental observations are compared with numerical simulations of fluid flows, is shedding light on the observable strokes, their efficiency, the underlying mechanisms, and metabolic requirements, with important consequences from the point of view of evolution. More generally, Mechanics is providing us with a conceptual framework to go beyond the identification of the molecular components and their individual function, by integrating them into a comprehensive understanding of cell motion and migration, of cell and tissue morphogenesis. The course will survey recent advances in the fields of cell motility and mechanobiology of cells and tissues, emphasizing such an integrative approach. Topics covered in the course will include the mechanics of cell motility by swimming and crawling, fluid-structure interaction problems in the modeling of individual and collective behavior of unicellular swimmers, synchronization effects, discrete and continuous models of the mechanics of the cytoskeleton, morphogenesis of cells and tissues and dynamics of their growth, mechanics of biological membranes and of active surfaces, design and engineering of bio-inspired motile artifacts. Both theoretical aspects (mathematical and computational modeling) and recent experimental findings will be surveyed. The Course will consist of 12 introductory lectures by the organizers, and 24 lectures on more specialized topics given by four among the most eminent experts in the field. The course is addressed to doctoral students, young researcher, senior researchers, practicing engineers and technologists.
D. Discher et al.: Tissue cells feel and respond to the stiffness of their substrate, Science 310 (2005), 1139-1143. R. Phillips et al.: Physical Biology of the Cell, Garland Science (2008). D. Fletcher and J. Theriot, An introduction to cell motility for the physical scientist, Phys. Biol. 1 (2004) T1–T10. F. Juelicher et al.: Generic theory of active polar gels: a paradigm for cytoskeletal dynamics, Europ. Phys. J. E 16 (2005), 5-16. R. Goldstein et al.: Direct measurement of the flow field around swimming microorganisms, Phys. Rev. Lett. 105 (2010), 168101-1-4. A. DeSimone et al.: Cytoskeletal actin networks in motile cells are critically self-organized systems synchronized by mechanical interactions, Proc. Nat. Acad. Sciences USA 108 (2011), 13978–13983. E. Paluch et al.: Actin cortex mechanics and cellular morphogenesis, Trends Cell Biol. 22 (2012), 536-545. M. Arroyo et al.: Reverse engineering the euglenid movement, Proc. Nat. Acad. Sciences USA 109 (2012), 17874–17879.
Marino Arroyo (None)
6 lectures on: Introduction to biological membranes and active surfaces. Lipid bilayer membranes: curvature elasticity; Dynamics of bilayer membranes: viscous dissipation; Dynamics of bilayer membranes: interlayer friction; Shape control of biomembranes; The euglenoid movement; Shape control by active surface deformation.Antonio De Simone (None)
6 lectures on: Introduction to cell motility by swimming and crawlin. Motile cells and mechanisms of cell motility; Low Reynolds number hydrodynamics and swimming at microscopic scales; Cilia and flagella; Actin-based motility; Protrusion and adhesion in cell crawling motility; Bio-inspired crawling motility in artificial systems.Daniel Fletcher (None)
6 lectures on: Building the cell: mechanics of cellular assembly. In vitro reconstitution of cellular structures; Force microscopy of the cytoskeleton; Mechanics of actin network assembly; Membrane-cytoskeleton interactions; Spatial organization of cell membranes; Mechanisms of membrane shape change.Jeffrey S. Guasto (Tufts University, Medford, MA, USA)
6 lectures on: The Physics of Multicellularity. Evolutionary Transitions to Multicellularity; Collective Behaviour in Microorganism Populations; Flagellar Synchronization I; Flagellar Synchronization II; Physics and Biology of Cytoplasmic Streaming I; Physics and Biology of Cytoplasmic Streaming II.Frank Jülicher (None)
6 lectures on: Active processes in cells and tissues. Introduction to molecular motors and the cytoskeleton; Dynamics of the cytoskeleton: active gels I; Dynamics of the cytoskeleton: active gels II; Biophyiscs of tissues; Tissue polarity and active tissue mechanics; Dynamics of tissue growth.Ewa Paluch (University College, London, UK)
6 lectures on: Cell morphogenesis. The shape of animal cells: the actin cortex; Actin cortex mechanics: flows, contractions, oscillations; The shape of migrating cells: protrusion formation, blebs versus lamellipodia; Mechanics of bleb formation; Migration in 3D environments: cortex flows and cell translocation; Protrusion formation, cell polarization and cell trajectories during 3D migration.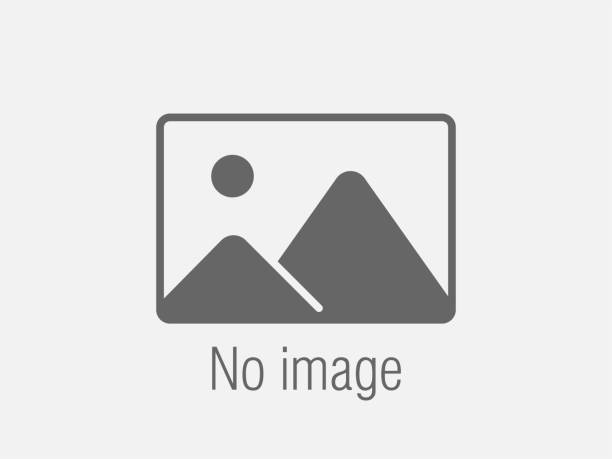