In the past two decades numerous laboratories have microfabricated surfaces with the chemical and textural properties to mimic superhydrophobic surfaces (SHs) found in nature, the most well-known being the self-cleaning properties of the lotus leaf. This has been made possible by the continuing advances in nano/micro fabrication technology. This Advanced School will bring together engineers, physicists, chemists and applied mathematicians in a multi-physics framework.
Adopting a holistic approach coupling momentum, heat, mass and charge transport phenomena the lecturers comprise 2 applied mathematicians, 2 mechanical engineers, a physicist and a chemist: together they bring theoretical and experimental perspectives to the topic.
The fundamentals of the physical and chemical phenomena exploited to suspend liquids in the Cassie (unwetted) state on SHs will be covered. We address the conditions required, and technologies developed, to maintain the Cassie state and those which cause transition to the (sometimes desirable) Wenzel (wetted) state. Transport phenomena physics related to droplets on SHs and flows of liquids over them will be emphasized.
For droplets on SHs, the fundamental microfabrication principles including those based on polymer processing technology will be surveyed. Ice prevention and enhancing boiling and condensation heat transfer will be points of emphasis, as will electrowettability-based dynamic control and enhancement of general phase change phenomena. Various approaches to suppress or exploit Leidenfrost phenomena to, e.g., suppress critical heat flux or pump droplets, will be studied. SHs with multifunctional properties such as photo-catalytic activity, anti-reflectivity, abrasion resistance and antisoiling characteristics will be treated.
In studying external/internal flows over SHs, the course will include a rigorous derivation of the governing equations and boundary conditions, resolution of surfactant, Marangoni, thermocapillary and molecular phenomena and possible meniscus deformations. Comparison of theoretical models to experiments will be made with implications for key engineering parameters.
The course is suitable for graduate students, academics, engineers in industry. The techniques used will span mathematical modelling ideas and numerical schemes, through to experimental procedures and understanding the fundamental physical principles. Applications will be emphasised throughout.
Game, S. E., Hodes, M., Keaveny, E. E., & Papageorgiou, D. T. (2017), Phys. Rev. Fluids, 2(9), 094102. Hodes, M., Kirk, T. L., Karamanis, G., & MacLachlan, S. (2017), J. Fluid Mech, 814, 301-324.
Krupenkin, T. N., Taylor, J. A., Wang, E. N., Kolodner, P., Hodes, M., & Salamon, T. R., (2007), Langmuir, 23(18), 9128-9133.
Kirk, T. L., Hodes, M., & Papageorgiou, D. T. (2017). J. Fluid Mech, 811, 315-349.
Crowdy, D.G. (2016), J. Fluid Mech, 791, R1.
Crowdy, D.G. (2017), J. Fluid Mech, 822, 307—326.
Philip, J. R. (1972), Z. Angew. Math. Phys, 23, 353-372.
Xu Q. F., Liu Y., Lin F-J, Mondal B., and Lyons A.M., (2013), ACS Appl. Mater. Interfaces, 2013, 5 (18), pp 8915–8924.
Pushalkar S., Ghosh G., Xu Q. F., Liu Y, Ghogare A.A., Atem C., Greer A., Saxena D., and Lyons, A.M., ACS Appl. Mater. Interfaces, (to appear). (DOI: 10.1021/acsami.8b09439).
Quéré D, (2013), Annual Review of Fluid Mechanics 45, 197–215.
Quéré D, (2008), Annual Review of Materials Research 38, 71–99.
Bahadur V. and Garimella S. V., (2008), Langmuir, 24, 8338-8345.
Bahadur V., Mishchenko L., Hatton B., Taylor, J. A., Aizenberg J., Krupenkin T. (2011), Langmuir, 27, 14143-14150.
Shahriari A., Birbarah P., Oh J., Miljkovic N. and Bahadur V. (2017), Nanoscale and Microscale Thermophysical Engineering (Special issue on Micro & Nanoscale Phase Change Heat Transfer), 1-20, 21(2), 102-121.
6 lectures on: Superhydrophobicity, electrowetting, microfabrication, heat transfer applications. Fundamentals of electrowettability on SHs. Ice prevention, the role of surface textures and chemistry in enhancing boiling and condensation heat transfer, electrowettability-based dynamic control and enhancement of phase change phenomena.
6 lectures on: Mathematical modelling of transport phenomena problems involving SHs and their applications. Complex variable, conformal mapping and transform techniques; techniques from asymptotic analysis and numerical methods. Ridge orientation, distribution, meniscus curvature, subphase fluid and non-Newtonian fluids effects on slip.
6 lectures on: Heat and mass transfer in internal flows through SH microchannels. Derivation of convective transfer equations for heat and species coupled to hydrodynamics. Thermocapillary stress and phase change along menisci. Gas diffusion in Cassie to Wenzel state transition. Analogies with thermal contact resistance. Cooling using liquid metals, novel pumping and propulsion mechanisms.
6 lectures on: Chemistry of SHs; effect of chemical and morphological properties on the stability and reliability of the Cassie state; fabricating mechanically durable and chemically stable SHs; fabricating SHs using polymer process technology; applications of SHs with multi-functional properties (e.g. photocatalytic activity, anti-reflectivity, abrasion resistance, anti-soiling).
6 lectures on: Hydrodynamics, including stability issues, of internal flows through SH microchannels; computation of meniscus location. Mathematical solutions for apparent slip lengths for range of problems capturing secondary effects such as surfactant-induced Marangoni stresses along menisci and edge effects. Numerical solutions, asymptotics and (spectral) Chebyshev collocation methods.
6 lectures on: Dynamics of liquids on structured surfaces. Super hydrophilic behavior: how to make liquid impregnate textures, how this generates low-adhesion surfaces, how a liquid inside a texture can be dislodged by another liquid. Water-repellency. Super aerophilicity. Leidenfrost with textures.
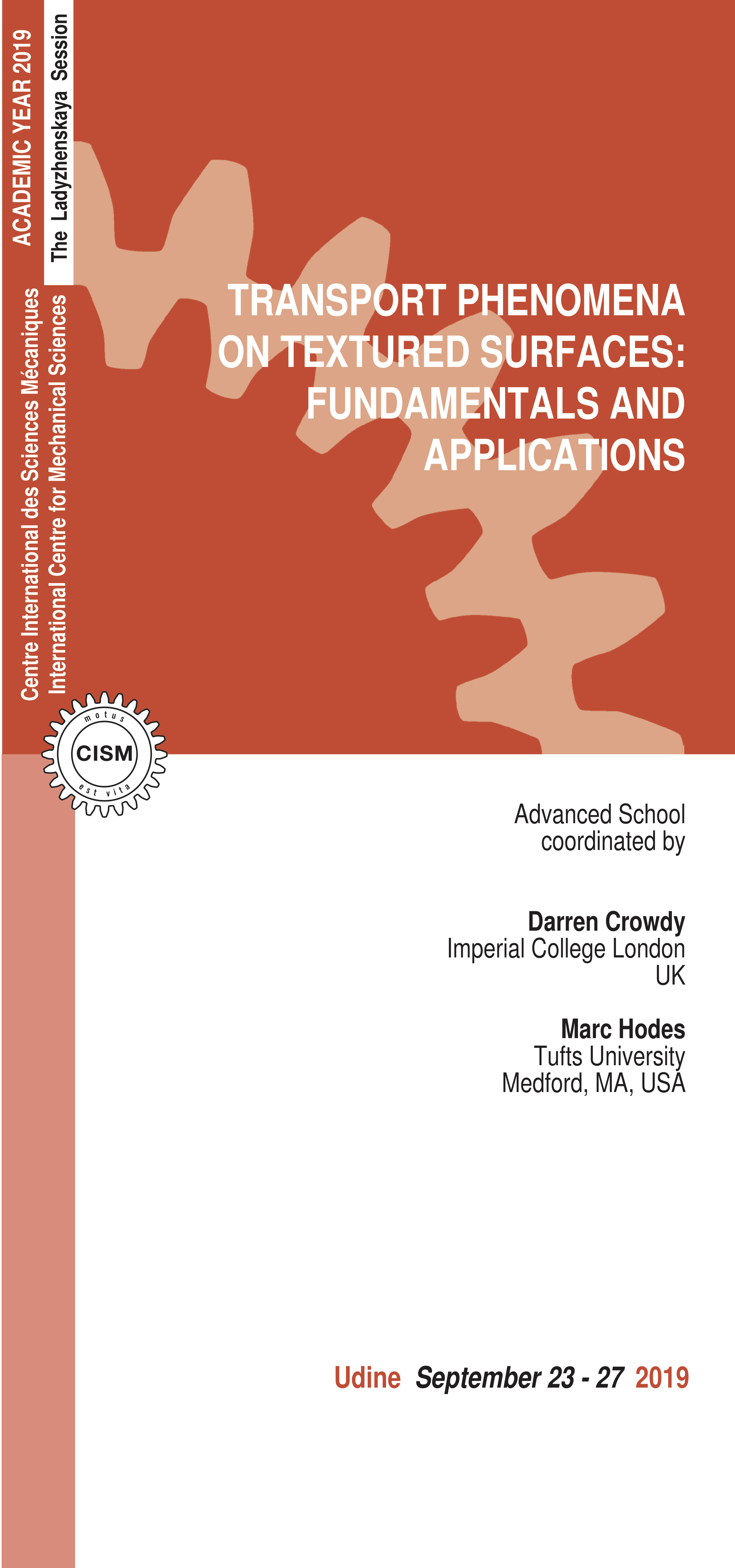