Understanding the dynamics of planetary and stellar fluid layers - including the atmosphere of the Earth and other planets, iron cores, and stellar convective and radiative zones – remains a tremendous interdisciplinary challenge, relying on common knowledge in fundamental fluid mechanics. A few of the numerous open questions include:
• What are the basic physical mechanisms responsible for climate circulation, and how can they be parameterised to reliably predict global climate change?
• What are the prevalent force balances and physical mechanisms behind natural large-scale features such as Jupiter's Great Red Spot and bands?
• What are the relevant driving forces and flow regimes in conducting planetary cores for explaining the generation of large-scale magnetic fields by dynamo action?
• How are the various types of waves propagating in the stellar interiors generated, what influence do they have on stellar evolution, and how can they be used to probe interiors via asteroseismology?
Interdisciplinary research in geo- and astrophysical fluid dynamics is also intrinsically multi-method. Indeed, the main obstacle to quantitative modeling and understanding of planetary and stellar flows stands in the extreme character of the involved dimensionless parameters. Relevant studies thus rely on the principle of dynamical similitude and scaling laws, sustained by theory, experiments and numerical simulations.
Much research effort has been devoted to understanding planetary and stellar flows within the various communities of Mechanics, Applied Mathematics, Engineering, Physics, Planetary, Atmospheric and Earth Sciences, and Astrophysics. But progress has mostly been confined to each separate domain, with only marginal cross-fertilisation. The objective of this school is to go beyond this state, by providing participants with a global introduction and an up-to-date overview of all relevant studies, fully addressing the wide range of involved disciplines and methods.
The course will be organized in three parts. The first will focus on fundamental aspects of fluid mechanics in geo- and astrophysical flows, including introductory material as well as current cuttingedge research, with a focus on instabilities, turbulence, and waves. The second part will focus on concrete applications to topical geo- and astrophysical problems, with lectures focusing on planetary interiors, atmospheres, and stars. Finally, the third part will involve practical numerical sessions using the open-source solver Dedalus (http://dedalusproject.org). Participants will learn to set up and run numerical simulations on their laptops related to the research problems discussed in the lectures.
The targeted audience for this school is PhD students, postdocs, and young researchers, working in departments of Mechanics, Applied Mathematics, Engineering, Physics, Planetary, Atmospheric and Earth Sciences, and Astrophysics. A background in fluid dynamics will be assumed for each participant, but no specific knowledge in any of the application domains or in computational methods will be requested. Each participant will be given the opportunity to present her/his work during a flash talk presentation, followed by a poster session.
Le Bars, Michael, and Daniel Lecoanet (eds). “Fluid Mechanics of Planets and Stars." Publisher: Springer (2020).
Vallis, Geoffrey K. "Atmospheric and Oceanic Fluid Dynamics." Publisher: Cambridge University Press (2006).
Sutherland, Bruce R. "Internal Gravity Waves." Publisher: Cambridge University Press (2010).
Mellado, Juan Pedro. "Cloud-top entrainment in stratocumulus clouds." Annual Review of Fluid Mechanics 49: 145-169 (2017).
Ogilvie, Gordon I. "Astrophysical fluid dynamics." Journal of Plasma Physics 82.3 (2016).
Davidson, Peter A. "Turbulence in rotating, stratified and electrically conducting fluids." Publisher: Cambridge University Press (2013).
Olson, Peter (ed). "Core Dynamics: Treatise on Geophysics." Publisher: Elsevier (2010).
5 lectures on:Stellar fluid dynamics, including: basics of stellar structure & evolution; stellar convection; the radiative-convective interface: overshoot, waves; angular momentum and chemical transport; asteroseismology.
5 practical work sessions: Participants will learn to set up and run numerical simulations using the Dedalus spectral solver on their laptops. Example simulations include: Rayleigh-Bénard convection; wave generation and propagation; wave mixing; solidification; moist convection.
5 lectures on: Fundamental fluid mechanics in rotating and/or stratified fluids relevant for geophysical and astrophysical flows, focusing on the most common instabilities (3 lectures) and on the basic properties of turbulence (2 lectures), including: barotropic and baroclinic instabilities; convection; geostrophic & wave turbulence; mixing.
6 lectures on: Fundamental fluid mechanics in rotating, stratified, and magnetized fluids, focusing on the generic properties of waves in geophysics and astrophysics (5 lectures), and on the basics of magneto-hydrodynamics (1 lecture), including: internal gravity waves; inertial waves; MHD waves; non-linear interactions; wave breaking; wave-mean-flow interactions; dynamo action.
5 lectures on: Atmospheric fluid dynamics, including: basic structure of the Earth’s atmosphere; planetary boundary layer; moist convection and clouds; inversion layer dynamics; wave-driven flows and instabilities.
5 lectures on:Internal planetary fluid dynamics, including: basics of planetary interior structure; convection and mixing in planetary cores and mantles; solidification in subsurface oceans; turbulence and dynamo action in planetary cores.
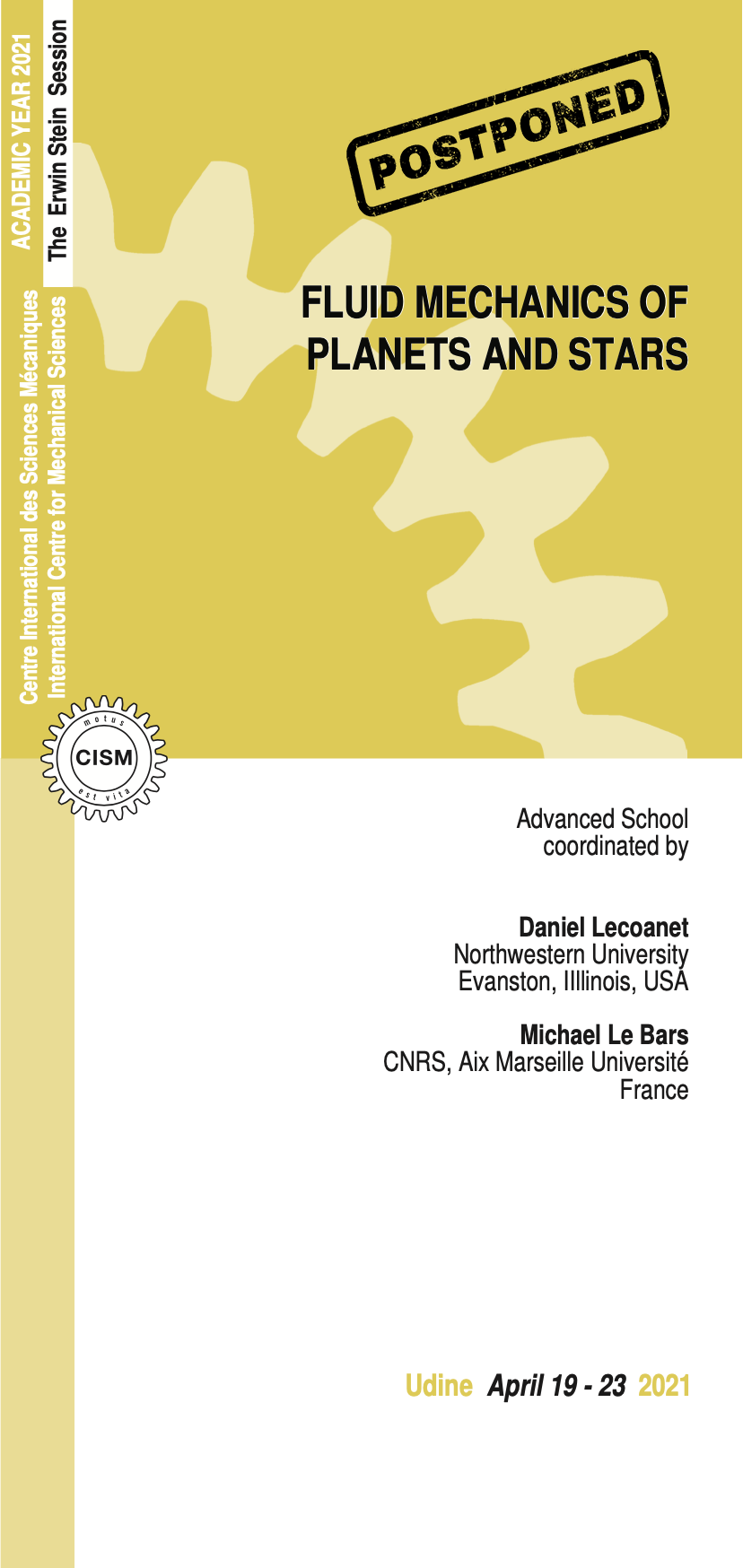